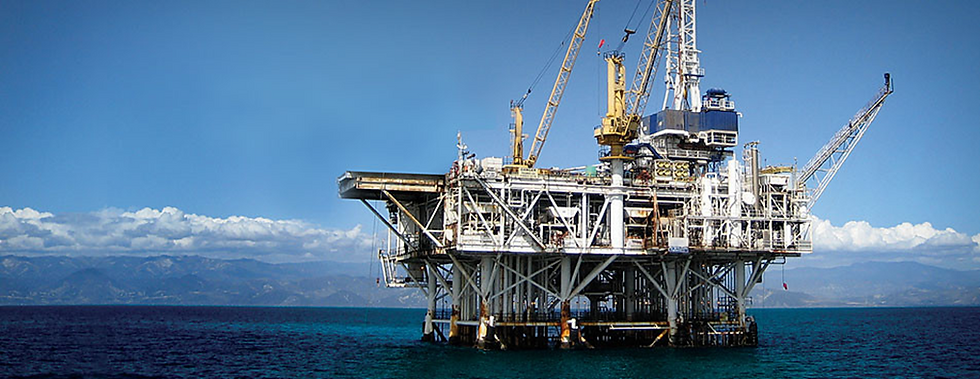
Nanotechnology is a field of advanced science and technology of governing matter on a nanoscale [1]. This nanoscale was first introduced in the famous lecture of Nobel Laureate Richard P. Feynman, “There’s Plenty of Room at the Bottom,” given in 1959 [2]. Nanomaterials have not only become one of the ‘hottest’ areas in research and development all over the world but also attracted numerous considerations in the industrial sectors [3][4]. This technology can be primarily defined by their functional properties which determine how they interact with other disciplines [5]. Recently, it becomes an evolving field in material science, materials processing technology, mechanics, electronics, optics, medicine, energy and aerospace, plastics and textiles etc. [6]. This technology not only establishes an interdisciplinary and emerging domain that embraces physics, chemistry, engineering [7] but also contributes to detection of diseases, better therapy options, and remarkable reduced health-care expenses [8].
Molecular nanomaterials can also be applied in manufacturing through ultra-precision, development of nano-metric microscopic devices, biological structures, nano robots, super computers, industries and genetics etc. [9]. All materials or devices which are nanometer scale (dimensions of roughly 1 to 100 nm) structured are included in nanomaterials [2][10]. Nanoscale, substances have a larger surface area to volume ratio than the bulk one which is the main reason for their increased level of reactivity, improved and size tunable magnetic, optical and electrical properties [11]. Nowadays, almost all developed nations have created nanomaterials-based research programs, fellowships, networks, research institutes, and educational enterprises aiming at understanding and leverage nanoscale discoveries [12][13].
Nanomaterials are now used in numerous industries all over the world. Nanofabrication, nanoparticles, nanorobot, nanocomputer, nanofertilizer, membrane-based nanoparticles, nano-engineered fiber, nanotubes, nanosensor, nanopesticides, nanoencapsulation, nano-ceramic tools, nanocatalysts etc. are used in industries to facilitate and develop improved industrial function and products. The structure in nanoparticles may rely on the method and conditions of particle preparation. The cohesive energies of the atoms inside nanoparticles and small clusters are additionally structure dependent [14]. When size approaches nanoscale the properties of particles change. The percentage of atoms becomes significant at the surface of nanoparticles, and there are numerous active sites on the nanoparticle surface.
Fabrication and Characterization of Molecular Nanomaterials
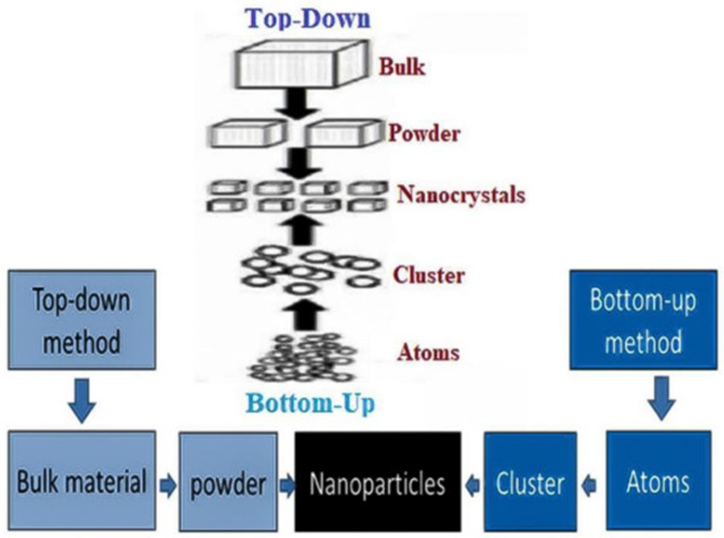
Nanoparticle production systems generate nanoscale particles in a precisely-defined, limited range of sizes for a range of uses, including the production of formulated coatings and other composite materials. To create the requisite size-dependent particle characteristics, a set of specific production and reaction parameters are required. Temperature, pH, chemical composition, surface modifications, and process variables may all be changed to choose the particle proportions, chemical make-up, crystallinity, and shape.[15]
Top-down and bottom-up manufacturing systems for nanoparticles are employed. Using a milling device to physically crush the raw material is referred to as "top-down" processing. Bottom-up techniques use chemical processes to create particles. The chemical composition and properties of the nanoparticles should be taken into consideration while selecting a technique.[16]
Several different approaches, including biological, physical, and chemical ones, are used in the creation of nanoparticles. Microorganisms including bacteria, fungus, and algae are utilized in biological processes to produce nanomaterials. In this instance, photosynthetic bacteria like Rhodopseudomonas capsulate may be used to extracellularly create gold nanoparticles that are 10–20 nm in size. In addition, extracellular silver nanoparticles may be created utilizing the Fusarium oxysporum fungus. In addition, within 12 hours of incubation, 95% of the gold nanoparticles were produced utilizing Sargassum wightii algae.
Silver nanoparticles may be created by employing a biomolecule found in orange (Citrus clementina) peel. Orange peel aqueous extract (OPE) and aqueous silver nitrate solution were combined at room temperature to create AgNPs. AgNP production was visible by the color shift from pale yellow to dark-reddish brown. Moreover, UV—visible spectroscopy was used at regular intervals in the wavelength range of 300–600 nm to examine the production of AgNPs spectroscopically[17].
In physical techniques, the top-down technique and bottom-up technique are two common methodologies that may be used to synthesize nanostructured materials. The size of the fundamental components utilized to construct nanomaterials with or without atomic level control makes a significant impact.
Moreover, nanoparticles are coated onto the surface of the completed product. In order to apply nanoparticles to textile fibers, a variety of coating processes, including sol-gel, plasma polymerization, and layer-by-layer, are utilized. These methods have the capacity to increase durability and make the cloth resistant to adverse weather. The surface texture of textiles can change depending on the mix of nanocoating ingredients, such as surfactants and carrier medium. [18]
Both the electrospinning technique and the split spinning procedure result in a nanofiber. Small fiber diameter, large surface area, high permeability, effective filtration, and thin layers are just a few of the many multifunctional qualities that nanofibers have. These materials have the capacity to filter dangerous chemicals, pathogens (bacteria, viruses), and poisonous gases from the air. The textiles gain toughness and abrasion resistance from the nanoparticles' uniform distribution in polymer matrix. The tensile strength of composite fibers can also be improved by nanofibers. A nanocomposite is a blend of two or more distinct nanometer-sized components. This method aids in the creation of textiles with enhanced biological, optical, mechanical, and electrical qualities. [18]
The method makes it possible to create computerized clothing, warming and cooling textiles, conductive textiles, communicative textiles, chromatic textiles, and textile sensors. Static electricity and metal ions are essential for giving fibers sterilizing characteristics. Lint, dog hair, and other items that are statically attracted are repelled by nano-based fibers thanks to their anti-static properties. Effective materials for reinforcing composite fibers include carbon nanoparticles and carbon nanofibers. [19]
For the creation of protective clothing for persons working in emergency services, such as military personnel, firemen, and medical staff, nanomaterials in textiles have shown to be of enormous use.There are different methods for the production of nanoengineered textiles. For example, sometimes synthesized nanoparticles are incorporated into the fibers or textiles.
General Uses:
· Antiwrinkle cotton fabric: For cotton fabrics, wrinkle resistance can be developed by using the nano-engineered cross-linking agents during the fabric finishing process. Besides the wrinkle resistance, such finishing is also capable of eliminating toxic gases, while preserving the preferred comfort properties of cotton.
· Odor-free fabric: Application of silver nanoparticles on fabrics prevent the nasty odor caused due to the microbial activity. Many companies use fabrics coated with silver nanoparticles to develop odor-free clothing, such as stockings, socks, and undergarments. Korean-based Hyosung develops nylon fibers containing silver nanoparticles that reduce 99.9% growth of several harmful bacteria.
· Water-resistant fabric: Silica nanoparticles create a water-resistant coating when inserted into the fabric or sprayed onto the fabric surface.
· Ultraviolet-protective fabric: When inserted into fabrics, nanoparticles of zinc oxide or titanium dioxide protect the skin from sun damage. These nanoparticles have the ability to scatter the ultraviolet light present in the sun’s rays, reducing the risk of skin diseases linked to UV exposure.
Is it Safe to Use Nanomaterials in Our Clothing?
Natalie von Goetz from the Swiss University ETH Zürich said that under laboratory conditions, no nanoparticles were found in the perspiration of the test person wearing fabric coated with titanium dioxide nanoparticles. She was responding to a frequently asked question about the safety of nanomaterials in our clothing. This is viewed as a successful outcome and is in favor of the use of nanoparticles in apparel.[15]
The issue with the inclusion of nanoparticles in textiles, however, is discussed by Rickard Arvidsson from Chalmers University of Technology in Gothenburg, Sweden. Although while silver nanoparticles are not dangerous to humans, he said, they may be extremely hazardous to aquatic life.
According to his research, the widespread usage of silver nanomaterial in garments might result in high silver concentrations in wastewater treatment plant sludge, which may ultimately have negative impacts on aquatic creatures. [14]
Construction Industry
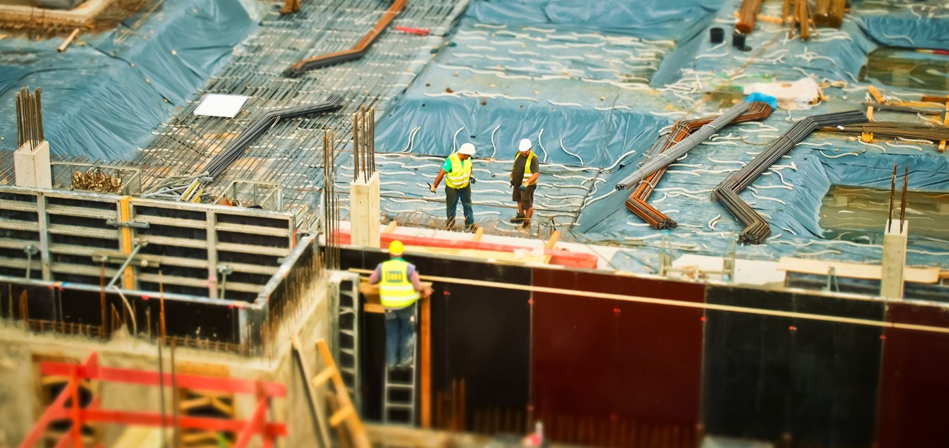
Nanomaterials can be categorized according to their dimension and composition. One-dimensional nanomaterials include thin films, layers, and surfaces; two-dimensional nanomaterials include nanowires and nanotubes; and three-dimensional nanomaterials include nanoparticles, graphite sheets, and quantum dots. Single phase solids, multiphase solids, and multiphase systems are the categories based on composition. Aerosols, colloids, and ferrofluids are examples of multiphase systems, while crystalline and amorphous particles, as well as layers, are examples of single phase solids. Multiphase solids also include matrix composites and coated particles.[20]
The creation of nanomaterials can be done in one of two ways: top down or bottom up. Whereas the bottom-up technique refers to building nanomaterials from atomic or molecular building blocks, the top-down approach refers to scaling down macroscale materials to nanoscale scales. [21]Nanomaterials can be produced using a single procedure or a combination of two processes. The top-down strategy, however, is frequently favoured since it is a much easier procedure.
The goal of nanotechnology is to create these nanoparticles and design them to create improved systems for applications that are affordable, sustainable, and effective. Nanotechnology advancements have an impact on most commercial sectors. Energy, electronics, food, agriculture, health care, cosmetics, and construction may all be added to the list.
The global economy of every country benefits greatly from the building sector. Nanotechnology has made less development than other industries, but it still has a lot of promise to help the building industry. It is possible to engineer traditional building materials like concrete, glass, structural steel, timber, and coatings to advance the sector and even support sustainable construction. The two most promising nanomaterials in the building industry are titanium dioxide (TiO2) and carbon nanotubes (CNTs). Clay, silica, alumina, magnesium, and calcium nanoparticles are other nanomaterials that can be employed in building. As they are not readily available in large numbers, nanomaterials like semiconducting films, quantum dots, and biomolecules are not useful to the building sector. Nanomaterials have considerable promise for the advancement of building materials due to their large surface area, high functional density, high sensitivity, unique surface effects, high strain resistance, and catalytic effects. Nanoparticulate fillers produce materials that are impermeable and improve mechanical characteristics. The choice of building materials, erection strategies, and on-site handling tactics are all directly impacted by improved material qualities. For instance, utilizing lighter materials in construction makes it simpler to erect structures; improving concrete placement time can shorten the construction timetable; and using products that are safer, easier, and faster to handle and install means requiring fewer workers overall.[22]
Nanotechnology for Concrete - One of the most vital components for the building sector is concrete. It readily contains additives, aggregates, and nanoscale structures like cement hydrates. The macroscale characteristics of concrete are significantly impacted by the features of these nanoscale formations. Engineering nanoscale characteristics can enhance the quality of the concrete. Comparing traditional structures used in building to nanostructures, traditional structures are less shear resistant, more flexible, and more ductile. Because of this, adding nanofiber reinforcements or particles to the structure boosts the endurance and strength of the concrete. For instance, carbon nanotubes increase the concrete's ability to withstand cracking. The characteristics of concrete may be improved by adding nanoparticles such as zirconium dioxide (ZrO2), nanotitanium oxide (TiO2), nanoiron (Fe2 O3), nanoalumina (Al2 O3), copper oxide (CuO), and zinc peroxide (ZnO2). Nano silica additions can enhance the self-compacting, segregation resistance, and durability of concrete.[21]
Self-leveling (SLC), self-healing, self-compacting, and ultra-high-performance concrete are all products of the use of nanofibers or nanoparticles (SCC). Moreover, certain nanotechnology applications used in the manufacture of cement promote sustainable building practices. A sizeable portion of CO2 emissions to the environment are caused by the cement producing process. Belite, calcium sulfo-aluminate, and calcium alumina-ferrite are a few additives that can lower the industrial process's CO2 emissions.
Nanotechnology for Structural Steel - Along with concrete, structural steel plays an important role in the construction industry. Nanotechnology is extensively used for the improvement of strength and durability of structural steel. Nano modifications such as material grain size refinement can reduce the cracking in steel structure. The use of carbon nanotubes increases the tensile strength of structural steel up to 150 fold while reducing the weight of steel by six times compared to the conventional steel structures in construction. Using lighter steel structures in the construction industry reduces the labor and energy requirements considerably. One of the most important issues of structural steel is fatigue. Fatigue results in structural failures upon cyclic loading and shortens the life cycle of the steel. Using copper nanoparticles can overcome this problem by creating smooth and even surfaces that reduce the stress on the steel structure. Nanotechnology can also be used for improving the corrosion resistance of steel by engineering new generation stainless steel materials.[21]
Nanotechnology for Coatings - Materials protection depends heavily on coatings, which nanotechnology can further enhance. Nanoparticles improve the chemical, optical, visual, and electrical qualities of coatings and paints as well as their resistance to corrosion and wear. They also stop condensation and mold growth. Nano-alumina and titania, for instance, exhibit four to six times more water resistance and two times greater strength. Moreover, these coatings can help keep structures safe and delay fires. In coatings that offer enhanced electrical and optical qualities as well as fire protection, carbon nanotubes are employed as harmless additions. Hard-surface coatings may be made with nano-TiO2 to resist wear and scratching as well as the adhesion of contaminants to building exteriors. Nano-silica particles are used in coatings to increase flexibility, gloss, and transparency without sacrificing surface flexibility, water resistance, scratch resistance, or abrasion resistance. Moreover, compared to the typical insulators used in construction, it is a more effective insulator. Aerogel coatings offer acoustic insulation, hydrophobic qualities, and resistance to mold, corrosion, and UV light-induced deterioration.[22]
The entire cost of construction, maintenance, and adjustments is decreased when nano-coatings are used. Moreover, nanotechnology can increase the thermal insulation of building materials, lowering carbon emissions and helping to conserve energy.
Nanotechnology for Timber - Forest products have gradually lost importance in the construction industry over the years due to their poor resistance properties in comparison to concrete and steel. However, nanotechnology can be utilized for the advancement of these bio-based materials. Carbon-based composites can provide strength and durability. Wood products are accessible for the construction industry; they are lightweight, easy to repair, and most importantly biodegradable.
Water Treatment Industry
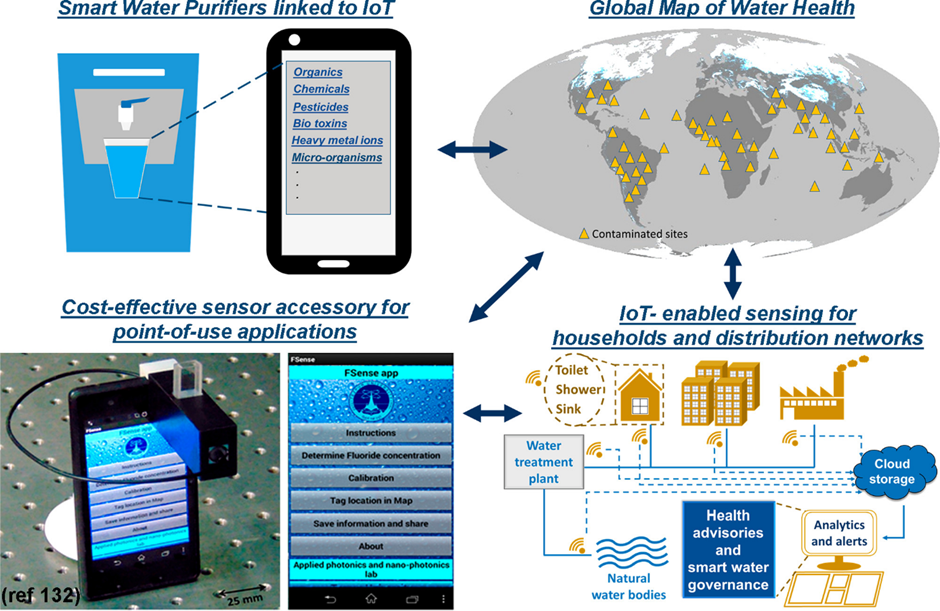
The countries, heavy rainfall can lead to erosion and soil run-off, which is why pathogens enter water bodies along with soil components and nutrients. Increasing air and raw water temperatures can have an impact on the cleanliness of drinking water pipelines and storage systems, leading to dangerous infectious diseases. For instance, legionella bacteria, which reach their peak population in warm water at 40°C, can cause Legionnaire's disease.[23]
Micropollutants, for example, are entering water bodies at an increasing rate in both industrialized and developing nations. [24]Traditional decontamination techniques like chlorination and ozonation use a lot of chemical agents and, in addition, might result in the production of harmful byproducts.
Advanced water and wastewater technology processes may now be developed thanks to the integration of extremely advanced nanotechnology with conventional process engineering.[25] An overview of current developments in nanotechnologies, including nanobased materials, processes, and their applications, is given here for water and wastewater processing. The constraints of nanotechnology for water applications, such as rules and regulations as well as possible health dangers, are discussed in addition to the hopeful scientific advancements.[24]
Due to their large specific surface area, discontinuous characteristics, and rapid dissolution, reactivity, and sorption, nanomaterials exhibit special size-dependent features (such as superparamagnetism, localized surface plasmon resonance, and quantum confinement effect).[23] These special nano-based properties enable the creation of innovative high-tech materials, such as membranes, adsorption materials, nanocatalysts, functionalized surfaces, coatings, and reagents, for more effective water and wastewater treatment processes.
Solids that are used to adsorb gases or dissolved substances are called adsorbents, and the adsorbed molecules are typically referred to collectively as the adsorbate. Adsorption is the ability of all solid substances to attract to their surfaces molecules of gases or solutions with which they are in close contact. 4 Nano adsorbents have a significantly greater rate of organic compound adsorption than granular or powdered activated carbon due to their large specific surface area.[25] They hold enormous promise for new decontamination techniques that are quicker, more effective, and remove both organic and inorganic contaminants, such as heavy metals and micropollutants. The higher process efficacy not only saves adsorbent materials but also makes it possible to employ smaller, more portable water and wastewater treatment equipment, especially for point-of-use and decentralized applications. Current research activities mainly focus on the following types of nanoadsorbents:
· carbon-based nanoadsorbents ie, carbon nanotubes (CNTs)
· metal-based nanoadsorbents
· polymeric nanoadsorbents
· zeolites.
When the consider about the Carbon nanotubes CNTs are allotropes of carbon with a cylindrical nanostructure. Depending on their manufacturing process, CNTs are categorized as single-walled nanotubes and multiwalled nanotubes, respectively. Besides having a high specific surface area, CNTs possess highly assessable adsorption sites and an adjustable surface chemistry. Due to their hydrophobic surface, CNTs have to be stabilized in aqueous suspension in order to avoid aggregation that reduces the active surface. They can be used for adsorption of persistent contaminants as well as to preconcentrate and detect contaminants.5 Metal ions are adsorbable by CNTs through electrostatic attraction and chemical bonding.[26]
Furthermore, CNTs exhibit antimicrobial properties by causing oxidative stress in bacteria and destroying the cell membranes. Although chemical oxidation occurs, no toxic byproducts are produced, which is an important advantage over conventional disinfection processes like chlorination and ozonation.7 They can be simply regenerated through appropriate adjustments of operating conditions, like pH shift.
When the consider about the Polymeric nanoadsorbents, Polymeric nanoadsorbents such as dendrimers (repetitively branched molecules) are utilizable for removing organics and heavy metals. Organic compounds can be adsorbed by the interior hydrophobic shells, whereas heavy metals can be adsorbed by the tailored exterior branches. integrated dendrimers in an ultrafiltration device in order to remove copper from water. Nearly all copper ions were recovered by use of this combined dendrimer-ultrafiltration system. The adsorbent is regenerated simply through a pH shift. However, due to the complex multistage synthesis of dendrimers, up until now there are no commercial suppliers, except for some recently founded companies in the People’s Republic of China.[26]
produced a highly efficient bioadsorbent for the removal of anionic compounds such as dye from textile wastewater by preparing a combined chitosan-dendrimer nanostructure. The bioadsorbent is biodegradable, biocompatible, and nontoxic. They achieve removal rates of certain dyes up to 99%.[27]
Magnetic nanoparticles - The use of magnetic nanoparticles (magnetite Fe3O4) for separation of water pollutants has already been established in ground water remediation, in particular for the removal of arsenic. The conventionally applied “pump-and-treat” technology for groundwater treatment comprises pumping up the groundwater to the surface and further treatment, usually by activated carbon for final purification. The considerably extended operating hours and higher environmental clean-up costs can be reduced by applying in situ technologies. Magnetic nanoparticles can be injected directly into the contaminated ground and loaded particles can be removed simply through a magnetic field. Besides ground water remediation, magnetic recovery makes such nanoparticles an ideal compound to increase the osmotic pressure of draw solutions used in forward osmosis. Forward osmosis as contrary process to reverse osmosis draws water from a low osmotic pressure to one with a higher osmotic pressure (draw solution) using the osmotic gradient.[28]
Nanosilver and nano-titanium dioxide - Nanosilver has been used in the photo development process since the late 1800s, and has been registered with the Environmental Protection Agency for use in swimming pool algaecides since 1954 and drinking water filters since the 1970s. Although nanosilver exhibits a strong and broad-spectrum antimicrobial activity, it has hardly any harmful effects in humans. It is already applied to point-of-use water disinfection systems and antibiofouling surfaces. Nano-titanium dioxide (TiO2), featuring high chemical stability and low human toxicity at a cheap price, is utilizable in disinfection and decontamination processes. Further information is provided in the section on photocatalysis. The main advantage of nano-TiO2 over nanosilver is the nearly endless life time of such coatings, since TiO2 as a catalyst remains unchanged during the degradation process of organic compounds and micro-organisms.[29] The antimicrobial effect of nanosilver is based on the continuous release of silver ions. After a certain operation period, depending on the thickness and composition of the nanosilver layers, the coating has to be renewed or the complete device, including the bulk material, has to be disposed of, leading to significant replacement costs. However, compared with TiO2, which needs energy-consuming ultraviolet lamps for activation, nanosilver kills bacteria with no need of additional energy-consuming devices. That makes nanosilver a favorable disinfectant for remote areas.[27][28]
A disadvantage of bactericidal nanoparticles in general except for nano-TiO2 is that no bactericidal substances such as hydroxyl radicals remain in the water past the contact time that could ensure the water quality in storage and distribution devices (depot effect).[30]
Oil and Gas Industry
Nanomaterials are used in the oil and gas sector to develop geothermal resources by increasing down-hole separation, thermal conductivity, and the development of noncorrosive materials. This might be applied to the generation of geothermal energy. The chances for exploiting unconventional and stranded gas resources might be enhanced by the use of nanomaterials. Many uses for nanotubes exist in the oil sector. [31]By making it easier to separate oil and gas in the reservoir for instance, by a better knowledge of processes at the molecular level nanoparticles may contribute to improving oil and gas output. By customizing surfactants, which can be supplied to the reservoir in a more controlled manner than with other compounds, nanomaterials have been used to increase oil recovery and release more oil.[32] The use of nanoparticles in oil reservoir engineering includes new kinds of smart fluids for drilling and better oil recovery. The upstream oil and gas business relies heavily on nanoparticles because they have unique qualities including adsorption, wettability, modification, and surface area to boost output. In the oil and gas business, nanoparticles are also used in drilling and completion processes. These processes include hydraulic fracturing, cementing, stabilizing clay, increasing drilling fluid viscosity and controlling fluid loss, controlling sloughing (wall collapse), and stabilizing well bores. Moreover, in the gas sector, air-suspended Ni-Fe nanoparticles are injected into the hydrate formation to pass through the voids and penetrate deep into the hydrate reservoir.[31][33] These nanoparticles can also cause the water cage to break down and release methane by raising the temperature by up to 42 °C and disrupting thermodynamic equilibrium. Lastly, by developing nanocatalysts, nanoscale membranes, and nanostructured materials for compressed natural gas (CNG) conveyance or long-distance power transmission, nanoparticles can solve the problems associated with accessing stranded natural gas deposits. CNTs are strong and effective as potential membrane building blocks for separating oil from water. As a result, the oil/water separation sector makes substantial use of CNT-based membranes. Nanomaterials are frequently employed in a variety of sectors to create new goods and improve the quality of those that hit the market quickly.[34]
References
1. Tamirat, Y. The Role of Nanotechnology in Semiconductor Industry: Review Article. J. Mater. Sci. Nanotechnol. 2017, 5, 202.
2. Sobolev, K.; Gutierrez, M.F. How Nanotechnology Can Change the Concrete World Successfully mimicking nature’s bottom-up construction processes is one of the most promising directions. Am. Ceram. Soc. Bull. 2021, 84, 15–17.
3. Zhu, W.; Bartos, P.; Porro, A. Application of nanotechnology in construction. Mater. Struct. 2004, 37, 649–658.
4. Havancsák, K. Nanotechnology at Present and its Promise for the Future. Mater. Sci. Forum 2003, 414, 85–94.
5. Silva, G. Neuroscience nanotechnology: Progress, opportunities and challenges. Nat. Rev. Neurosci. 2006, 7, 65–74.
6. Asif, A.K.M.A.H.; Hasan, M.Z. Application of Nanotechnology in Modern Textiles: A Review. Int. J. Curr. Eng. Technol. 2018, 8, 227–231.
7. Muñoz-Écija, T.; Vargas-Quesada, B.; Chinchilla Rodríguez, Z. Coping with methods for delineating emerging fields: Nanoscience and nanotechnology as a case study. J. Inf. 2019, 13, 100976.
8. Satalkar, P.; Elger, B.; Shaw, D. Defining Nano, Nanotechnology and Nanomedicine: Why Should It Matter? Sci. Eng. Ethics 2015, 22, 1255–1276.
9. Bale, A.S.; Aditya Khatokar, J.A.; Singh, S.; Bharath, G.; Kiran Mohan, M.K.; Reddy, S.V.; Satheesha, T.; Huddar, S.A. Nanosciences fostering cross domain engineering applications. Mater. Today Proc. 2021, 43, 3428–3431.
10. Sawhney, A.P.S.; Condon, B.; Singh, K.V.; Pang, S.S.; Li, G.; Hui, D. Modern Applications of Nanotechnology in Textiles. Text. Res. J. 2008, 78, 731–739.
11. Nigam Joshi, P. Green Chemistry for Nanotechnology: Opportunities and Future Challenges. Res. Rev. J. Chem. 2016, 5, 3–4.
12. Grieneisen, M.; Zhang, M. Nanoscience and Nanotechnology: Evolving Definitions and Growing Footprint on the Scientific Landscape. Small 2011, 7, 2836–2839.
13. Mulvaney, P.; Weiss, P. Have Nanoscience and Nanotechnology Delivered? ACS Nano 2016, 10, 7225–7226.
14. Deng, L.; Hu, W.; Deng, H.; Xiao, S. Surface Segregation and Structural Features of Bimetallic Au−Pt Nanoparticles. J. Phys. Chem. C 2010, 114, 11026–11032.
15. Bereznenko, S., Bereznenko, N., Skyba, M., et al. (2020) A novel equipment for making nanocomposites for investigating the antimicrobial properties of nanotextiles. International Journal of Clothing Science and Technology. Vol. Ahead of Print. Available at: https://doi.org/10.1108/IJCST-07-2019-0107
16. Mahmud, R. and Nabi, F. (2017). Application of Nanotechnology in the field of Textile. Journal of Polymer and Textile Engineering. 4 (1), 1-6. Available at: http://www.iosrjournals.org/iosr-jpte/papers/Vol4-Issue1/A04010106.pdf
17. Nanalyze (2018) 7 Startups Innovating in Nano Clothing Technologies. [Online] Available at: https://www.nanalyze.com/2018/02/7-startups-nano-clothing-technologies/ (Accessed on 28 May 2020).
18. Saratale, R.G.; Shin, H.S.; Kumar, G.; Benelli, G.; Ghodake, G.S.; Jiang, Y.Y.; Kim, D.S.; Saratale, G.D. Exploiting fruit byproducts for eco-friendly nanosynthesis: Citrus × clementina peel extract mediated fabrication of silver nanoparticles with high efficacy against microbial pathogens and rat glial tumor C6 cells. Environ. Sci. Pollut. Res. 2018, 25, 10250–10263.
19. Kolahalam, L.A.; Kasi Viswanath, I.V.; Diwakar, B.S.; Govindh, B.; Reddy, V.; Murthy, Y.L.N. Review on nanomaterials: Synthesis and applications. Mater. Today Proc. 2019, 18, 2182–2190.
20. Kashef, M.; Sabouni, A.R. Nanotechnology and the building industry. In Proceedings of the International Conference on Nanotechnology: Fundamentals and Applications, Ottawa, ON, Canada, 4–6 August 2010; pp. 550–558.
21. Du, S.; Wu, J.; Alshareedah, O.; Shi, X. Nanotechnology in cement-based materials: A review of durability, modeling, and advanced characterization. Nanomaterials 2019, 9, 1213.
22. Eftekhari, M.; Mohammadi, S.; Khanmohammadi, M. A hierarchical nano to macro multiscale analysis of monotonic behavior of concrete columns made of CNT-reinforced cement composite. Constr. Build. Mater. 2018, 175, 134–143.
23. Choi, Y.C. Cyclic heating and mechanical properties of CNT reinforced cement composite. Compos. Struct. 2021, 256, 113104.
24. Jayapalan, A.R.; Lee, B.Y.; Kurtis, K.E. Effect of Nano-sized Titanium Dioxide on Early Age Hydration of Portland Cement. In Nanotechnology in Construction 3; Springer: Berlin/Heidelberg, Germany, 2009; pp. 267–273.
25. Yaqoob, A.A.; Parveen, T.; Umar, K.; Mohamad Ibrahim, M.N. Role of Nanomaterials in the Treatment of Wastewater: A Review. Water 2020, 12, 495.
26. Alvarez, P.J.J.; Chan, C.K.; Elimelech, M.; Halas, N.J.; Villagrán, D. Emerging opportunities for nanotechnology to enhance water security. Nat. Nanotechnol. 2018, 13, 634–641.
27. Wang, Z.; Wang, Z.; Lin, S.; Jin, H.; Gao, S.; Zhu, Y.; Jin, J. Nanoparticle-templated nanofiltration membranes for ultrahigh performance desalination. Nat. Commun. 2018, 9, 2004.
28. Jassby, D.; Cath, T.Y.; Buisson, H. The role of nanotechnology in industrial water treatment. Nat. Nanotechnol. 2018, 13, 670–672.
29. Danwittayakula, S.; Jaisaib, M.; Duttab, J. Efficient solar photocatalytic degradation of textile wastewater usingZnO/ZTO composites. Appl. Catal. B Environ. 2015, 163, 1–8.
30. Qian, C.; Yin, J.; Zhao, J.; Li, X.; Wang, S.; Bai, Z.; Jiao, T. Facile preparation and highly efficient photodegradation performances of self-assembled Artemia eggshell-ZnO nanocomposites for wastewater treatment. Colloids Surf. A Physicochem. Eng. Asp. 2021, 610, 125752.
31. Nabhani, N.; Emami, M.; Moghadam, A.B.T. Application of nanotechnology and nanomaterials in oil and gas industry. AIP Conf. Proc. 2011, 1415, 128–131.
32. Ponmani, S.; Nagarajan, R.; Sangwai, J. Applications of nanotechnology for upstream oil and gas industry. J. Nano Res. 2013, 24, 7–15.
33. Singh, N.A. Nanotechnology innovations, industrial applications and patents. Environ. Chem. Lett. 2017, 15, 185–191.
34. Saththasivam, J.; Yiming, W.; Wang, K.; Jin, J.; Liu, Z. A Novel Architecture for Carbon Nanotube Membranes towards Fast and Efficient Oil/water Separation. Sci. Rep. 2018, 8, 7418.
Comments